High Energy Laser Directed Energy WeaponsTechnical Report APA-TR-2008-0501 | ||||||||||||
| ||||||||||||
(Images RuMoD, Almaz, US Air Force, US DoD)
![]() YAL-1A ABL prototype in flight (U.S. Air Force) | ||||||||||||
| ||||||||||||
Introduction
Directed Energy Weapons (DEW) have been a recurring theme in science fiction literature and cinema ever since H.G. Wells published the 'War of the Worlds' in 1898. The idea of a 'death ray' which can instantly destroy or burn a target at a distance retains its allure to this very day. More than a century after Wells contrived his 'heat ray', the technology is maturing to the point of becoming soon deployable.
High Energy Laser weapons have been progressively evolving since the 1960s, a path punctuated by a series of important scientific breakthroughs and engineering milestones. The popular view of a HEL, seen as constructing a great big laser and pointing it at a target with the intention of vapourising it, bears only vague similarity to a real HEL weapon. There are genuine technological and operational challenges involved in creating truly useful and effective weapons. Kinetic or projectile weapons such as guns, missiles and bombs destroy targets by kinetic effects, including overpressure, projectile, shrapnel and spalling damage, and incendiary effects. The result is structural damage and fire, which can and often will cause fatal damage to a target. A kinetic weapon thus uses stored chemical energy in propellants and warhead explosives, the latter where used, and delivers this energy to a target by means of a projectile of some kind. Whether the projectile weapon is a trebuchet tossing a large rock over 300 yards, or a multimode seeker equipped long range air to air missile hitting an aircraft from 200 nautical miles away, the underpinning principle is much the same, only the implementation is different. At the most fundamental level Directed Energy Weapons share the concept of delivering a large amount of stored energy from the weapon to the target, to produce structural and incendiary damage effects. The fundamental difference is that a Directed Energy Weapon delivers its effect at the speed of light, rather than supersonic or subsonic speeds typical of projectile weapons. Two of the most fundamental problems seen with projectile weapons, taht is getting the projectile to successfully travel a useful distance and hit the target, and then produce useful damage effects, are problems shared by Directed Energy Weapons. Having a powerful laser or microwave emitter maketh not a Directed Energy Weapon system alone. Most contemporary literature lumps together a broad mix of weapons technologies in the Directed Energy Weapon category, including High Energy Laser (HEL) weapons, High Power Microwave (HPM) weapons, particle beam weapons and Laser Induced Plasma Channel (LIPC) weapons. The first two of these four classes of weapon are genuine Directed Energy Weapons. Particle beam weapons are best described as a form of projectile weapon, using atomic or subatomic particles as projectiles, accelerated to relativistic speeds. The LIPC is a hybrid, which uses a laser to ionise a path of molecules to the target, via which an electric charge can be delivered into the target to cause damage effects. Of these four categories, HELs have the greatest potential in the near term to produce significant effect. HPM technology has similar potential, but has not been funded as generously and thus lags well behind lasers. LIPC has significant potential especially as a nonlethal weapon. Particle beam weapons at this time are apt to remain in the science fiction domain, as the weight and cost as yet do not justify the achievable military effect. The next ten years will see the emergence of high energy lasers as an operational capability in US service. These weapons will have the unique capability to attack targets at the speed of light and are likely to significantly impair the effectiveness of many weapon types, especially ballistic weapons. Constrained by propagation physics, these weapons will not provide all weather capabilities, and will perform best in clear sky dry air conditions. | ||||||||||||
Early High Energy Laser EvolutionThe connection between laser and microwave weapons runs deep, both in terms of the physics and the evolutionary history of these technologies. The basic physics of the Maser and the Laser are the same. Dr Charles Townes, who codiscovered the Maser (Microwave Amplification by Stimulated Emission of Radiation) in 1953 at Columbia University, later collaborated with Dr Arthur Schawlow at Bell Labs to create, in 1958, the first Laser (Light Amplification by Stimulated Emission of Radiation). Both Lasers and Masers act as amplifiers of electromagnetic radiation and, if equipped with mirrors to bounce this radiation back and worth inside the device, can act as oscillators and thus sources of electromagnetic radiation. The inner workings of both devices rely on an phenomenon called 'stimulated emission' whereby an atom or molecule which has been excited to a given energy level, will emit that energy as a photon in the visible light or microwave bands, if it is hit by another photon with exactly that energy level. If you can excite a volume of gas or other material, within which a large proportion of atoms or molecules are of a specific type, with specific energy levels, shooting a single photon of that energy level into the volume will see a cascade effect, with a vastly larger number of like photons coming out the other end. In practical terms such a device converts the energy used to excite the material, into a stream of light or microwave photons of a specific wavelength or colour. The process of exciting the device is termed 'pumping'. Pumping can be achieved in a variety of ways, using light (flashlamps or other so called 'pump' lasers), electrical discharges (in gas lasers), electrical current (semiconductor lasers) or shockwaves in gas flows (Gas Dynamic Lasers or Chemical Lasers). The earliest lasers of interest involved the use of crystal rods, for instance made of ruby, or discharges in argon or carbon dioxide gas. Because lasers produced light which was coherent and almost spectrally pure, that light could be easily focussed. By the mid 1960s researchers were producing many kiloWatts of power and burning holes into plates of metal and other materials. Unfortunately crystal rod lasers and gas discharge tube lasers suffer a common problem, which is power conversion efficiency, typically at best several percent. As a result most of the power put in to pump the device is converted into waste heat. A period example is a gas discharge laser with a total length of 60 metres producing a mere 9 kiloWatts of power, yet wasting at least ten times as much. ![]() ![]() ![]() Researchers needed a laser technology which was suitable for generating hundreds of kiloWatts or MegaWatts of power, and which had a conversion efficiency in the tens of percent, before any weapons application was feasible. In the early sixties a number of physicists suggested it may be possible to pump a molecular gas to laser action by rapid heating or cooling. Further research showed that such cooling could be achieved through the expansion of a heated gas through a supersonic nozzle. In 1966, a team of physicists and engineers working for Avco Everett constructed and operated the world's first Gas Dynamic Laser (GDL), operating on a mixture of C02, N2 and H20. By 1970, continuous power outputs of 60 kiloWatts were being generated and a 1973 pulsed GDL delivered 400 kiloWatts for 4 milliseconds. It was then clear that High Energy Laser weapons were feasible. This technology was the basis of the subsequent US Air Force Airborne Laser Laboratory and underpins the current chemical laser technology used in the YAL-1A ABL system. Gas Dynamic Lasers, now termed Chemical Lasers (CL), bear in some respects more similarity to rocket engines than the commonly popularised rod laser. A laser propellant, comprising a suitable mix of chemicals, is combusted or reacted and the exhaust efflux is then directed into an expansion nozzle. The exhaust stream from the expansion nozzle contains highly energetic molecules, which due to the choice of propellants and added agents, have effectively been pumped to a state where laser action can occur. If a pair of aligned mirrors is placed to either side of the exhaust stream, laser action will occur as photons bounce between the mirrors, and power can be extracted if one of the mirrors is optically leaky. While simple in principle, the design and construction of a Chemical Laser is anything but trivial. Combusters operate at temperatures as high as 1000 to 2000 deg C, depending on the laser fuel mix used. The expansion nozzles require very precisely controlled flow conditions to work, which results in a complex exhaust system designed to produce the required pressure and flow rates. Some laser fuels and/or their exhaust efflux can be highly corrosive and/or toxic. Mirrors must have very low optical losses, since even a 1 percent loss in a 1 MegaWatt laser sees 10 kiloWatts of waste heat dumped into the mirrors. The first chemical lasers built used carbon monoxide (CO) burned in oxygen-nitrogen, with water being added, to produce the same 10.6 micron band laser action used in carbon dioxide gas discharge lasers. CO burning in N20 and benzene (C6H6) burning N20 were also explored as fuels. While a single laser, comprising a combustor, expansion nozzle and exhaust duct could produce respectable power levels, it was clear that many such devices needed to be cascaded to produce power levels suitable for weapons applications. This is why all contemporary Chemical Lasers use batteries of smaller lasers to produce the final high power output beam. While the carbon dioxide laser was the first in this class, it was soon followed by the Hydrogen Fluorine (HF), Deuterium Fluorine (DF) and Oxygen Iodine (COIL) lasers. The HF laser uses atomic fluorine and molecular hydrogen to produce 2.7 - 2.9 micron band radiation, using typical fuels such as highly toxic SF6 or NF3, with hydrocarbons used to produce hydrogen. Its later sibling, the DF laser, uses ethylene (C2H4) burned with a nitrogen trifluoride (NF3) oxidiser, into which deuterium and helium are injected, to produce 3.6 to 4.2 micron band radiation. The most significant of these three discoveries was the Chemical Oxygen Iodine Laser (COIL), invented by the US Air Force Weapons Laboratory in 1977, and now used in the YAL-1A system. The COIL emits in the 1.315 micrometer range, and uses chlorine gas and an aqueous mixture of hydrogen peroxide and potassium hydroxide to produce excited oxygen molecules, which are reacted with molecular iodine to produced the laser medium, and which is then passed through the expansion nozzles. Conversion efficiencies above 20% were demonstrated very early. ![]()
Chemical Oxygen Iodine Laser
As is clearly evident, building a large laser may be the basis of a HEL weapon, but a considerable amount of hardware will be required to actually put it to use. ![]() | ||||||||||||
Peace Through Light - the Airborne Laser Lab Program![]() By the early 1970s it was clear to the US DoD that an airborne laser weapon was feasible using existing laser technology, an idea which was actively promoted during the late 1960s by physicist Dr Edward Teller, co-inventor of the hydrogen bomb. This led to a series of experiments during the 1970s to demonstrate viability and identify problems. The first was in 1973 when the USAF shot down a winged drone at their Sandia Optical Range, New Mexico, using a carbon dioxide GDL and a gimballed telescope. Subsequently, in 1976, the US Army employed an electrically pumped HEL to destroy a number of winged and helicopter drones at the Redstone Arsenal in Alabama. The USN, in March 1978, then engaged and destroyed an Army TOW missile in flight, using a chemical laser developed by DARPA/USN and a pointer-tracker developed by the USN. These tests were carried out at San Juan Capistrano near Camp Pendleton in California, as part of the Unified Navy Field Test Program. The US Air Force launched their Airborne Laser Lab (ALL) program in 1976, under the motto 'Peace Through Light'. The aim of this effort was to construct a technology demonstrator, carried on modified NKC-135 Stratotanker serial number 55-3123, which could successfully track and destroy airborne targets. The ALL system used a gas dynamic laser with CO2 - N2 - H2O propellants and a 10.6 micron operating wavelength. Pratt & Whitney Aircraft supplied the laser, Rocketdyne the combustor, Hughes the optical pointing and tracking system, Perkin Elmer the dynamic alignment system, with GD performing system integration. ![]() The ALL system was complex enough to fill a KC-135A fuselage, with the HEL weapon located in the forward and centre fuselage areas, and test crew in the aft fuselage (USAF). ![]() The ALL laser system combustors operated at 55 atmospheres of pressure, and at a 1900 K temperature, driving nickel plated nozzles with an output velocity of Mach 6. The optical resonator used one concave and one convex mirror, with beam extraction via an aerodynamic window, produced using high pressure nitrogen gas, with the efflux at 0.1 atmospheres of pressure. The ventral exhaust port, forward of the wing root under bomb bay like doors, used a corrugated titanium diffuser and vented gas at 870 K temperature, delivering a thrust of 4,000 lbf when operating. The supporting fuel system stored CO and N2O gas in stainless steel tanks, liquid Helium and Nitrogen in stainless steel tanks, with additional water coolant tanks for the mirror and combustors. This laser produced a raw output of 456 kiloWatts of optical power in an 8 second sustained run, and an output from the optical aiming system of 380 kiloWatts, reported in 1979 literature. At a distance of 1 kilometre, the delivered power density was over 100 Watts/cm2. The ALL project ran for eleven years, culminating in a series of trials during which five AIM-9 missiles were shot down, and a single BQM-34A Firebee drone destroyed. While the ALL attracted much media attention as the world's first airborne laser weapon, its real value was in the enormous amount learned during the program. Several key problems rose to prominance. The first was the issue of power losses in the optical feed, tracking and beam pointing system. Not only did waste heat have the potential to wreak havoc inside the system, but dust particles inside the system when hit by the intense infrared beam would be propelled at high velocity, as they partly vapourised, and damage optical surfaces. Cleanroom air quality was required. It also became very evident that high precision target tracking equipment was needed, and the whole beam pointing system required extremely low jitter when tracking a target. The aim was to put a 'football sized' spot on the target and 'dwell' the beam for long enough to burn through the skin of the target and cause serious damage. Jitter in beam pointing significantly reduced effect, the problem getting worse with increasing distance. ![]() This image shows a technician working on the optical telescope turret of the ALL HEL weapon. Note the array of ancillary optical sensors (USAF). Beam propagation through the atmosphere presented anticipated and unanticipated problems. Water vapour molecules, water droplets and carbon dioxide molecules soaked up the beam, causing localised heating along the beam path which caused the beam to dissipate. This effect was termed 'thermal blooming' and would become more severe as beam power levels increased. A general limitation of all HEL weapons is an inability to penetrate cloud, dust clouds or haze, which scatter and soak up the beam's power very rapidly. Target damage effects were another issue. Not only did the HEL laser beam have to operate at a wavelength which would experience a minimum of absorption by atmospheric molecules, but it also had to be capable of rapid absorption by structural materials making up the skin of the target. Aluminium for instance has around 98 percent reflectance for the 10.6 micron CO2 laser. ![]()
NKC-135A Airborne Laser Laboratory (USAF).
![]() Other problems arose as a result of scintillation due to turbulence in the atmosphere, which is characteristically made up of pockets of air with slightly differing temperature. Slight temperature differences mean slight air density differences, which would cause the beam to refract (bend) every so slightly as it passed between two pockets of air. With thousands of such distortions along the beam path, this problem presented a difficult obstacle to achieving useful range, especially during low altitude operations, where the beam would be severely defocussed along the way. The ALL NKC-135A was retired in 1984, and sent to the Wright-Patterson Air Force Museum in 1988. | ||||||||||||
The Star Wars Breakthrough – Adaptive MirrorsThe much maligned Reagan era Strategic Defense Initiative or 'Star Wars' program, intended to drive the Soviets to bankruptcy, yielded one very important dividend for the world of HEL weapons – the adaptive mirror. At the time of the ALL experiments, solutions were in sight for most if not all of the practical problems encountered with HEL weapons. Lasers could be scaled, optics made larger, tracking systems more accurate and longer ranging. The problem of penetrating the turbulent and thermally inhomogenous atmosphere was not solved. To penetrate the atmosphere without defocussing beam distortion, the beam itself would have to be 'pre-distorted' as it leaves the optics of the HEL weapon, so that the wavefront of the beam arrived at the target undistorted and precisely focussed. While this is a simple idea in concept, it is harder in practice, as the motion of the HEL platform, motion of the target and movement of the air mass force the need for the pre-distortion of the beam to change continuously. Any solution thus has to include apparatus for continuously measuring the distortion along the path to the target, and a mechanism to continuously distort the HEL beam. Two technologies were developed to solve this problem. One is the adaptive or deformable mirror which has up to hundreds or thousands of miniature actuators, each of which can locally raise or depress the surface of the mirror, to distort the beam in a controlled fashion. These 'rubber mirrors' are capable of distorting the wavefront in a controlled fashion, with enough accuracy to compensate for atmospheric problems. The second technology is a form of Lidar (laser radar) which is used to continuously measure the distortion along the beam path to the target. These systems will illuminate the target with a lower power laser operating at a wavelength which is similar but not identical to the HEL weapon. This laser illumination is backscattered off the target and then fed into a device called a wavefront sensor, which measures the distortion across the whole cross section of the beam path to the target. The most commonly used device, the Hartmann-Shack wavefront sensor, uses an array of tiny 'lenslets' placed infront of an imaging device like a CCD. If the wavefront is perfectly flat, a dot appears centred beneath each of the lenslets. If part of the wavefront is distorted over the position of a lenselet, the dot's position moves in a manner specific to the direction and size of the distortion. ![]()
Hartmann Shack Wavefront Sensor (Kiepenheuer-Institut für Sonnenphysik)
In a HEL weapon system, the Lidar and wavefront sensor are used to continuously measure the distortion along the beam path, and produce correponding commands to the actuator array used in the deformable main mirror, which reflects the high power HEL beam to the target. | ||||||||||||
![]() The AL-1A Airborne Laser (ABL)![]()
(U.S. Air Force)
The solution of the beam distortion problem paved the way for an operationally viable HEL weapon system. At the end of the Cold War the SDI program was quietly killed off, but some key proposals survived. The Airborne Laser (ABL), envisaged as a follow-on to the ALL program, but with an operational role, was one of these. In 1996, the US Air Force awarded a US$1.1 billion contract to Boeing, TRW and Lockheed-Martin to develop a prototype ABL system, to be carried in a Boeing 747-400 aircraft. The ABL was to use a MegaWatt class COIL HEL weapon and a system to compensate for atmospheric distortion, to permit boost phase attacks on ballistic missiles. A single ABL system would thus defend a footprint of hundreds of kilometres diameter, attacking and destroying launched ballistic missiles during their boost phase, when they are most detectable, slowest and most vulnerable due to heavy fuel load, pressurised fuel tanks and structural stresses. Ballistic missiles have thin load bearing skins, which are heavily stressed during the boost phase, while the missile boosters are largely filled with pressurised high energy propellants. Therefore even slight damage to the booster skin will cause catastrophic failure with results seen many times over during failed launches of satellite launch vehicles. ![]()
Boeing/TRW/LM YAL-1A Airborne Laser Concept (USAF)
The ABL would be deployed in time of crisis to the borders of a nation threatening the use of ballistic missiles, and should they be launched, destroy them, ensuring that debris with WMD warheads falls on the launching party. At the time the ABL was conceived, these nations included Iraq, Iran and North Korea. With ongoing growth in Iran's and North Korea's arsenals, and their efforts to deploy nuclear warheads, the ABL could prove to be a vital asset if either of these nations achieves their aims. One of the design aims of the ABL system was to carry enough laser fuel to destroy twenty to forty missiles during a single 12 to 18 hour sortie. The ABL would orbit near the border of a threat nation and engage missiles as soon as they clear the cloudbase and are within line of sight. The capabilities of the ABL system have raised the prospect of another operational application, which is the Anti-Satellite (ASAT) role. In this role, the AL-1A would fly an intercept profile to intersect the ground track of a low orbit reconnaissance or surveillance satellite, or manned space vehicle, and damage or destroy it. While satellites are more robust structurally than ballistic missiles, the ABL delivers more power over the same distance when attacking an orbital target, due to the much lower atmospheric density along the beam path, compared to an atmospheric target. Satellites are also equipped with sensitive optics and vulnerable solar panels. Suffice to say even public debate on this application elicited loud complaints from non-US operators of military satellites. | ||||||||||||
In a crisis the ABL systems would be deployed to the borders of a nation threatening a ballistic missile attack, good candidates now being Iran and the DPRK. Orbiting at the tropopause, the ABL would detect, track, and attack ballistic missiles once they clear the cloudbase, with the debris falling back on the nation which launched the weapon. The latter is a critical consideration for WMD payloads. A design objective for the ABL is to carry enough fuel to destroy 20-40 missiles during an 12 to 18 hour sortie. Other roles canvassed for the ABL include attacks on low orbit reconnaissance satellites. The airframe used to carry the ABL system is the YAL-1A which is a modified Boeing 747-400F freighter. This is a robust and mature 100 tonne payload class airframe, with a main deck which is comparable to the C-5 Galaxy in payload and capacity. ![]() The YAL-1A ABL will be the first large laser weapon to become operational. Its purpose is to destroy ballistic missiles during the boost phase (Boeing). ![]() The most notable external feature of the AL-1A is the nose mounted optical turret for the laser's primary mirror. The turret has a +/-120 degree field of regard in azimuth and is used to point the 1.6 metre primary laser mirror, produced by Corning Glass and Contraves. The roll shell is constructed from composite materials. When the laser is not in use, the 1.8 metre 150 kg window, built by Heraeus/Corning/Contraves, is rotated into a stowed position to protect the optical surface from abrasion by atmospheric dust particles, and birdstrike damage. The main deck forward of the wing is separated from the aft main deck, which houses the laser system, by a full height bulkhead. The forward fuselage section houses the Battle Management system and the Beam Control Subsystem. The Battle Management System (BMS) comprises the computers which manage the weapon system, the operator consoles for the weapon system, and supporting communications. Built around open systems COTS hardware and software, the system is the nerve centre of the ABL. It performs the identification, tracking, determines the priority and nomination of targets, and controls the engagement. To do this, the battle management system relies on offboard sensors and an onboard infrared tracking and rangefinding sensor. The latter is a derivative of the legacy LANTIRN targeting pod, using the existing longwave FLIR sensor to tracking the missile, and a new carbon dioxide 10.6 micron band rangefinding laser and sensor, to supplement angle track data with accurate range. The intent is to produce an accurate trajectory projection for the target missile to facilitate 'prioritising' targets for attack. The sensor is mounted in a dorsal pod, carryied on a short pylon. The third subsystem in the forward fuselage is the Beam Control System (BCS). This is the critical component which ensures that the laser's power can be effectively delivered to the target. The BCS comprises the wavefront sensor and control system for beam distortion control, the systems for beam jitter control, beam alignment and beam 'walk' control, calibration hardware, and target acquisition and tracking equipment. The deformable mirror has 341 actuators which update the shape of the mirror at 1,000 Hz frequency – this means 1/1000 sec is required not only to measure the distortion but also to calculate and control the mirror actuator. ![]() The lower forward cargo hold is retained and intented for carrying equipment to support deployments. The aft main deck area carries the HEL subsystem and supporting hardware. Immediately aft of the wing are the two supporting lasers, built by Raytheon and Northrop-Grumman. These are the Tracking Illuminator Laser (TIL) and Beacon Illuminator Laser (BIL), both diode pumped solid state devices. The TIL is used to illuminate the target to facilitate fine tracking, while the BIL is used to measure atmospheric distortion to compensate beam wavefront shape, via the wavefront sensor.
![]() Aft of these are the main laser power stages, using 1.315 micron band COIL technology. Plastics, composites and titanium are used extensively to save structural weight. Despite this, each of the six laser modules weight around 1.5 tonnes. Each of the modules vents exhaust efflux via six ventral exhaust ducts and ports (see photo). The laser stages are complex. The gaseous atomic oxygen fuel for the COIL is produced in a reactor which mixes Helium (He) hydrogen peroxide liquid (H2O2) and potassium hydroxide (KOH) producing waste heat and potassium chloride (KCl). The hydrogen peroxide is recycled in a closed loop system until it has been exhausted. US sources claim that 1,200 USG of propellant is to be carried. The atomic Oxygen produced is then mixed with gaseous Iodine (I), to produce the excited Iodine required for laser operation. The gaseous mix is then flowed through a supersonic expansion nozzle, which also acts as the laser cavity. ![]()
Ventral aft fuselage exhaust ports for the COIL laser.
The high power beam is flowed through all six laser stages gaining power with each stage, for an aggregate output of the order of a MegaWatt of continuous wave power. The full power beam is directed via a system of mirrors to the beam control subsystem and then the optical turret in the nose. In an operational environment the AL-1A would be positioned into an orbit near enough to cover the territory of interest and loiter awaiting target tracks. Surveillance systems such as orbital early warning satellites, AEW&C where equipped with suitable radar, and UAVs would detect the initial launch of the missiles, relaying this data via Link-16 to the AL-1A. Once the ABL system is cued, the aircraft positions for a shot - if required the aircraft will turn to face the threat sector to afford the best possible field of regard for the laser weapon. As the ballistic missiles break through the overcast, their enormous heat signatures would be detected by the LANTIRN sensor and coarse tracking initated. The BMS would attempt to establish missile trajectories as early as possible to determine priority for engagement. While the US Air Force have not disclosed how they intend to do this, it is reasonable to speculate that the value of the target and the distance to the missile would both be factors in the beam scheduling algorithm. Not unlike phased array radars used for missile defence, there is a finite time window to attack each target and a finite amount of laser time available within this window. Therefore judicious scheduling of laser use is essential to provide opportunities for reattack if needed. More distant targets will require that the laser 'dwell' on the target much longer to achieve effect, compared to nearer targets. Once a coarse track for a specific missile has been established and the laser scheduled to shoot, the turret is slewed to point at the target and the TIL illuminator is lit up to initiate fine tracking. Once the fine track is established, the BIL beacon is lit up to generate continuous data on atmospheric distortion along the beam path. With the BIL operating the COIL laser is engaged and a multi-MegaWatt beam of 1.315 micron infrared radiation is put on to the target. In a viable engagement scenario, this would lead, within seconds, to the breakup of the target missile. Once this has happened, the system is cued to the second highest priority target and the same engagement sequence repeated. This would continue until laser fuel is exhausted or all of the targets killed, or out of reach. What defences exist against the ABL? Given the signature size of a ballistic missile, detection and tracking is unavoidable once the missile clears any cloud. At that point the only defence lies in improving the missile's resistance to laser attack. Years ago a physicist remarked to this author that this was 'simple, you cover the missile with a mirror coating to reflect the laser'. This is of course easier said than done, since any dirt, dust, moisture droplets, ice particles or other material on the surface of even a perfect mirror will vapourise and the superheated plasma will eat into the surface, destroying its reflectivity. At best mirror surfaces increase required laser dwell time to destroy the target. Another strategy proposed has been to impart a rotation to the missile, effectively causing it to spin around its longitudinal axis, to minimise local exposure time to the laser, the idea being that through the remainder of each rotation the skin would cool. But this strategy also at best delays the inevitable, and could at best impact required dwell time. A third strategy proposed has been the use of ablative surface coatings which would evaporate and so both cool the surface and block the beam in a layer of superheated vapour. A variation on this theme is the use of highly heat resistant skin materials. Both of these strategies would significantly add to the cost and weight of a missile, impacting deployable numbers and useful warhead size. A fourth strategy proposed has been the use of higher rocket thrust per payload so the missile climbs out of the atmosphere in a much shorter time, thus reducing firing opportunities for the laser. If the missile can complete its boost pahse in half the time, the time available for attack is halved, which during a massed launch would allow some fraction of missiles to get past the laser. As with the preceding strategy, cost/numbers becomes a major issue since a much bigger missile first and second stage is required for the same warload. Let us assume that a player develops a fast burn, spinning ballistic missile with an ablative coating, covered by a mirror coating. What it means is that more ABL platforms will need to patrol a given area to ensure that the increased dwell time does not allow any missiles to escape. The attacker will have to spend a lot more on his missiles. Like all missile defence technologies, the ABL drives up the cost of mounting a successful missile attack, to the point where it may not be economically viable. Suffice to say the intensive interest of China and Iran in cruise missiles indicates that the ABL, and ground based interceptor missiles, are already having impact well before they have even achieved full operational capability. The ABL program has been controversial, to say the least. As it is directly competing for funds with capabilities such as interceptor missiles, be they silo, warship or air launched, there is an added element to the controversy, as all players attempt to maximise their slice of the budgetary pie. The COIL laser achieved 'First Light' in November, 2004, with an initial test run. However, significant integration and testing remains before the system will be viable for operational use. This February the buy of five production airframes was put on hold, until such time as the capabilities of the prototype could be proven. Current planning envisages a trial shot against a target ballistic missile in late 2008. Recent US reports indicate that many problems remain to be resolved. One is that of atmospheric dust particles in the main beam, termed 'fireflies'. Given the intensity of the beam, dust particles vapourise and the plasma exacerbates local turbulence and soaks up energy from the beam, reducing effective range. It also has the potential of interfering with the fine tracking function, which relies on infrared reflections off the target. There has been considerable speculation on the use of the ABL for other roles, excluding the previously mentioned ASAT role. One idea has been to use the ABL to attack cruise missiles. If these are high flying supersonic weapons like the Kh-22 Burya/Kitchen flying a relatively flat trajectory, then the ABL will be highly effective. If they are low flying cruise missiles in the class of the Tomahawk or ALCM, then effectiveness is apt to be poor. The same is true of low flying aircraft targets or surface targets. The reality is that the troposphere, below 36,000 ft, is a poor propagation environment with a lot of water vapour and dust particles, or water droplets in cloud. The tropospheric 'soup' absorbs and dissipates the energy in the laser beam a lot faster than the dry/cold/thin stratosphere does. Physics are physics and cannot be easily beaten. The result will be very poor effective range, and an unusuable weapon if any cloud gets between the laser and the target. Much the same constraints apply if the target is an aircraft. A high flying UAV, reconnaissance aircraft or even hypersonic vehicle is extremely vulnerable to the ABL. A low flying aircraft is not. In perspective the AL-1A ABL is a revolutionary weapon which once mature will render ineffective arsenals of short, intermediate and intercontinental ballistic missiles, and high flying aircraft and cruise missiles, where conditions permit the ABL to operate within lethal range of the target. How soon the ABL matures into an operationally viable system remains to be seen. ![]() The Tactical High Energy Laser (THEL)![]()
US Army Tactical High Energy Laser (THEL) beam director turret.
The THEL is a laser weapon jointly developed by the US and Israel, with the program initiated in 1996. The THEL is to be built in two configurations, the static baseline THEL and relocatable Mobile THEL (MTHEL). The design aim of the THEL systems is to provide a point defence weapon which is capable of engaging and destroying artiellery rockets (Katyushas), artillery shells, mortar rounds and low flying aircraft. The THEL demonstrator was trialled repeatedly between 2000 and 2004, destroying 28 122 mm and 160 mm Katyusha rockets, multiple artillery shells, and mortar rounds, including a salvo attack by mortar. The demonstrator THEL system was built around a deuterium fluoride chemical laser operating at 3.8 microns wavelenght. The combustor in this laser burns ethylene in toxic and corrosive Nitrogen Trifluoride gas to produce the excited deuterium fluoride lasing medium, which is then mixed with deuterium and helium, and fed into expansion nozzles similar to that of other chemical lasers, like the carbon dioxide GDL and COIL. A complex exhaust diffusion and pressure equalisation system must be used, including a neutralisation stage to soak up the highly corrosive and toxic deuterium fluoride exhaust efflux gas. The first deuterium fluoride laser to be trialled was the US Navy's MIRACL, or Mid-Infrared Advanced Chemical Laser, which was coupled to the Sea Lite Beam Director optical turret. This research system was trialled extensively since 1983 at the High Energy Laser Systems Test Facility (HELSTF Directorate) at White Sands in New Mexico. This was a MegaWatt class weapon. The THEL program yielded excellent trial results, using a phased array radar to track incoming targets and direct the beam. Unlike the ABL, the THEL is a relatively short range weapon used for the terminal defence of a local area, not unlike a point defence SAM or AAA system. ![]()
The Sea Lite beam director was coupled to the MIRACL deuterium fluoride laser, demostrating the technology later used in the THEL demonstrator (US Navy).
![]()
THEL optical turret subsystem. Note the cassegrainian primary aperture (US Army).
![]() A 160 mm Katyusha artillery rocket engaged by the THEL system. ![]() ![]() THEL/MTHEL phased array engagement radar. ![]() THEL engagement scenario. ![]() MTHEL is a repackaged and mobile derivative of the THEL demonstrator. ![]() MTHEL diagram from patent application by Northrop Grumman. The sheer bulk of the demonstrator made it impractical for operational deployment, leading to the second generation MTHEL system. MTHEL was to initially have been in three semitrailers, but now appears to have been repackaged into a single container sized semitralier. A prototype was intended to be deployed by 2007, but more recent reports indicate funding difficulties and thus uncertainties in timelines. The THEL/MTHEL system was developed by a team including TRW/Northrop-Grumman, Ball Aerospace, Elbit/El-Op, IAI/Elta who developed the radar and fire control system, RAFAEL and Tadiran. Like the ABL, the THEL/MTHEL suffers the same weather and propagation limitations imposed by atmospheric physics. While Israel, with dry desert climatic conditions may be optimal for THEL operations, the same is not apt to be true for humid tropical environments, or northern temperate environments, where moist air, fog, low cloud and similar propagation impediments are much more common. In operational terms a key limitation of this design lies in the expensive and toxic exotic fuel mix. At several thousand dollars per shot in fuel expenditure, the Deuterium Fluoride laser is problematic for a system to be deployed en masse. In strategic terms the impact of the THEL is yet to be determined, unlike the ABL which is set to change the game fundamentally. The current MTHEL development effort is sustained and yields a viable product, it is apt to become a feature of ground based air defence over the coming decade. At the time of writing funding had been stopped for this effort, the focus shifting to electrically pumped solid state laser technology. High Energy Laser Technology Demonstrator![]() The High Energy Laser Technology Demonstrator (HEL TD) is a follow on effort by the US Army to demonstrate a mobile multi-hundred kiloWatt class laser using solid state laser technology. The budget justification for the effort, running until 2013, is thus: "A. Mission Description and Budget Item Justification: This applied research program element (PE) investigates advanced technologies for Future Force High Energy Laser (HEL) weapons technology, and, where feasible, exploits opportunities to enhance Current Force capabilities. The major effort under this PE is the development of a multi-hundred kilowatt (kW) Solid State Laser (SSL) laboratory demonstrator that can be integrated into a HEL weapon system to provide increased ground platform-based lethality. HEL systems have the potential to address the following identified Army capability gaps: 1) Defeat In-Flight Projectiles such as rockets, artillery, mortars, anti-tank guided missiles, and man-portable surface-to-air missiles; 2) Ultra-Precision Strike with little to no collateral damage; 3) Disruption of Electro-Optical (EO) and Infra-Red (IR) sensors; and 4) Neutralizing mines and other ordnance from a stand-off distance. HELs are expected to complement conventional offensive and defensive weapons at a lower cost-per-shot than current systems. At weapon system power levels of greater than 100kW, SSL technology has the potential to enhance survivability by addressing the capability gaps identified above. This SSL technology effort addresses technical issues such as high average power output from compact and more efficient lasers; precision optical pointing and tracking; laser effects degradation due to atmospheric effects; lethality against a variety of targets; and effectiveness against low-cost laser countermeasures. The multi-hundred kilowatt laser and additional HEL technology components will be refined and upgraded to transition into an integrated SSL weapons system demonstrator that will be developed in PE 0603004A (Weapons and Munitions Advanced Technology) Project L96. Project NA5 funds congressional special interest items. Work in this PE is related to, and fully coordinated with, efforts in PE 0602890F and PE 0603924F (High Energy Laser Joint Technology Office), PE 0605605A (DoD High Energy Laser Systems Test Facility), and PE 0603004 (Weapons and Munitions Advanced Technology) Project L96. The cited work is consistent with Strategic Planning Guidance, the Army Science and Technology Master Plan (ASTMP), the Army Modernization Plan, and the Defense Technology Area Plan (DTAP). Work is performed by the U.S. Army Space and Missile Defense Command (SMDC), in Huntsville, AL, and the High Energy Laser Systems Test Facility, White Sands Missile Range, NM." Northrop-Grumman and Boeing have been contracted for this effort. Boeing will integrate the beam control subsystem on a Heavy Expanded Mobility Tactical Truck (HEMTT). | ||||||||||||
Advanced Tactical Laser (ATL)![]() The ATL program is an effort to exploit the technology developed for the ABL to provide a cheaper and smaller system, suitable for carriage on aircraft such as the AC-130 Spectre or V-22 Osprey, as a close air support weapon. In January 2006, U.S. Air Force's 46th Test Wing provided Boeing with a C-130H Hercules for trials of the prototype weapon, claimed to be in the MegaWatt class, using COIL technology. The intent was to mount the laser prototype in the aircraft and perform lethality trials in 2007 against a range of ground targets. L-3 Communications/Brashear developed the optical turret, and the laser is being built by AFRL at Kirtland Air Force Base in Albuquerque, New Mexico. The laser was successfully fired in May, 2008. ![]() The Solid State High Energy LaserAn alternative HEL technology now under development is the solid state electrically pumped laser, popularised extensively in 2002 as part of the Joint Strike Fighter marketing campaign. This category of laser is based on the humble laser diode, which first emerged twenty years ago as a technology for use in optical fibre communications. Laser diodes are evolved siblings of the common Light Emitting Diode (LED), found in household appliances and now even battery powered torches. Light emitting diodes, usually made from Gallium based alloys, generate light as a result of electrical current flow through a minute semiconductor junction, which has been doped with additives which become excited by the electrical conditions in the junction. An LED is an incoherent and relatively broad emitter, compared to a laser. Current LED technology spans colours from infrared through to blue. The distinction between an LED and Laser Diode is that the latter is fabricated with a minature optical resonator, to induce oscillation and thus laser action. To narrow the bandwidth of the cavity, most communications laser now also include a diffraction grating in the design, in addition to the resonant cavity. A simple description of a laser diode is that is a special type of LED, designed to exhibit laser action. At this time Laser diodes are manufactured in vast quantities for CD and DVD burners, and also for communications and industrial applications. The solid state laser effort led by the US Air Force at the Air Force Research Lab aims to develop a 25 kiloWatt weapon, scalable to 100 kiloWatts, and suitable for carriage by aircraft, ground vehicles and ships as a close in weapon. The attraction of this technology is that electrical power is easy to supply, and toxic propellants are not required. The idea behind the these high power lasers is to couple together a very large number of much smaller laser diode modules, which are then use to pump the laser medium with energy. This a essentially a scaled up equivalent to the laser dioded pumped 1.55 micron eyesafe rangefinding lasers now in use. The principal problems currently faced with this technology are cost, scalability and power handling capability. Conversion efficiency from electrical power to optical output for these systems is typically of the order of 10%, which means that 90% of the electrical power put into the laser is converted into waste heat, which needs to be removed. The individual laser diodes are also not very powerful, typically emitting around one Watt each. A laser rated at 25 kW with a 10% conversion efficiency would require 250,000 diodes. At $10 per diode, this results in a multimillion dollar investment in lasers alone. Public reports on this program largely vanished over the last three years, suggesting it is no longer funded. Joint High Power Solid State Laser
The JHPSSL effort is intended to develop a family of electrically power high power solid state lasers suitable for 100 kiloWatt class weapons applications, primarily for land andd sea based point defence systems. The JHPSSL program is jointly funded by the Army Space and Missile Defense Command, Huntsville, Alabama; Office of the Secretary of Defense - Joint Technology Office, in Albuquerque, New Mexico; the Air Force Research Laboratory at Kirtland Air Force Base; and the Office of Naval Research in Arlington, Virginia.
![]() Northrop Grumman demonstrated early 2008 a 15.3 kiloWatt laser module for this program (refer image). Textron systems have been contracted to produce a competing JHPSSL design, using proprietary ThinZag laser ceramic Neodymium doped Yttrium-Aluminum-Garnet (Nd:YAG) slab technology. High Energy Liquid Laser Area Defense SystemThe High Energy Liquid Laser Area Defense System (HELLADS) effort was launched following a research breakthrough by DARPA, in 2003, with a budget of around US$75M. Its aims were described by the US DoD thus: 'The goal of the High Energy Liquid Laser Area Defense System (HELLADS) program is to develop a high-energy laser weapon system (~150 kW) with an order of magnitude reduction in weight compared to existing laser systems. With a weight goal of less than 5 kg/kW, HELLADS will enable high-energy lasers (HELs) to be integrated onto tactical aircraft and will significantly increase engagement ranges compared to ground-based systems. The HELLADS program has completed design of a revolutionary high energy laser that supports the goal of a lightweight and compact high energy laser weapon system. An objective system laser module with integrated power and thermal management will be fabricated and demonstrated at an output power of 15 kW. Based on the results of this demonstration, additional laser modules will be developed and integrated with a beam control subsystem to produce a 150 kW laser weapon system demonstrator. The performance of the demonstrator will be characterized in a laboratory environment. Program Plans: − Develop and test a 15 kW objective system laser module with integrated power and thermal management subsystems. − Complete preliminary design of a 150 kW laser weapon system. − Complete detailed design and fabricate a 150 kW laser weapon system. − Demonstrate performance of a 150 kW HEL system.' In practical terms, HELLADS is to produce a 750 kg laser capable of producing 150 killoWatts of power, compact enough to be carried by aircraft, ground vehicles and UAVs. The technology is a liquid laser, where the lasing medium is a fluid containing the active chemical species pumped for laser action. No details of the design concept have been released so far, but it is reasonable to speculate that the liquid is actively cooled thus avoiding the problems inherent in solid state lasers. The pump mechanism has not been disclosed. If pumping is performed using laser diodes, it is reasonable to speculate that the liquid also cools the pump lasers. General Atomics is the prime contractor, with Lockheed-Martin providing integration. HELLADS will like the ABL use a wavefront sensor and active mirror technology for beam wavefront correction. A demonstration is planned for 2007. High Power Fibre Laser (HPFL) - Optical Fibre Laser TechnologyOptical fibre lasers are a recent technological development, devised to provide low noise amplification for long haul submarine optical fibre communications systems. The technology is currently being developed further for weapons applications by Raytheon, following a demonstration conducted with the AFRL and Sandia in June, 2006.The iompetus for the3 development of fibre lasers lay in reducing the cost and complexity of repeater amplifiers for fibre cable links, especially for rapidly evolving, complex high speed waveforms. Eliminating complex and inflexible electro-optical repeaters in links allow costs to be significantly improved and the longevity of a cable installation significantly extended. Performance upgrades in many instances will require only the replacement of the line terminal equipment at either end of the link, rather than the chain of repeaters. By the early 1980 a race was underway in the fibre research community to find suitable laser technology for adaptation to the unique fibre environment. Early research explored Raman effect amplification and adaptations of established semiconductor lasers. Both proved to be disappointing, with Raman effect designs requiring unreasonably large pumping power due to their low efficiency, and semiconductor lasers having high signal distortion. The breakthrough came in the mid 1980s when a research group in Southampton in the UK devised a rare earth ion doping technique for silica fibres, building on the same 1960s research which led to the now standard military Nd:YAG laser, used for bomb guidance. The first Erbium Doped Fibre Amplifier (EDFA) paper was published in 1987. The next important development was the 1.48 micron InGaAsP laser diode, the pump power source required to optically excite the Erbium ions embedded in the glass, to amplify at 1.55 microns. Japan's NTT published their results in 1989. With a compact and efficient pump source and doped fibre technology the practical EDFA became a reality. To build one, the starting point is a spool of Erbium doped optical fibre of a suitable length. The input, where the EDFA is coupled to the end of a fibre link, uses a wavelength selective coupler. The coupler is used to feed the flow of pumping photons from the pump laser into the EDFA. These photons propagate along the fibre, exciting it. The "signal" photons pass through the coupler into the EDFA, and are amplified in number as they pass through the excited fibre. When they reach the output end of the spool, they are fed into an optical splitter. Most of the photons go into the next segment of the fibre link, but some are split off to feed a local optical detector. The electrical output from the detector is then used in a negative feedback loop to control the power level produced by the pump laser. In this manner the EDFA gain can be quite precisely controlled. While conceptually the EDFA is fairly simple, the laser physics and system design issues can be quite complex. Commercially available EDFA technology at this time covers a wide range of packaging and performance specifications, and a number of different pumping wavelengths and pumping designs. Current designs commonly employ a two stage arrangement, using silica glass, combinations of silica glass and fluoride glass, or fluoride glass fibres alone. Noise figures for communications fibre lasers typically vary between 4.5 and 9 dB (competitive with GaAs electrical receivers), gains between 25 and 40 dB, and output power levels between 13 and 20 dBs. Around 2000 commercial designs optimised for 1.3 micron systems, using praseodymium doped fluoride fibre amplifier (PDFFA) technology, emerged, achieving similar performance to 1.55 micron EDFAs. ![]()
The fundamental physics of a High Power Fibre Laser (HPFL) do not differ from a communications fibre laser. The differences lie in power levels, and where applicable, operating wavelengths, typically in the 1.5 micron to 1.8 micron range.
US research effort in this area aims to produce laser power outputs of the order of a 100 kiloWatts or more, which is suitable for point defence weapon applications. To achieve this, power per fibre must be increased, and optical hardware to combine the ouput of multiple HPFLs is required. Effective cooling of pump lasers and the HPFL spools is also required. The attraction of HPFLs is in their exploitation of mature commercial technologies, compactness, and electrical pumping compared to chemical lasers. Raytheon Laser Area Defense System (LADS)![]() Raytheon's LADS is intended to provide a short range point defence weapon as a replacement for the Phalanx CIWS, utilising existing Phalanx hardware and systems. Cite: "Raytheon has completed a series of activities that culminated in an early demonstration of LADS. The LADS unit consisted of an Air Force Research Laboratory (AFRL) 20-kilowatt IPG Photonics fiber laser and a bench-mounted beam director secured to the top of a Phalanx mount. AFRL testing verified the ability to propagate the laser beam and achieve the desired effect for a 60 mm mortar in a real-world environment. Sandia National Laboratories provided explosive test chambers, targets and an outdoor range for live-fire testing of LADS against operational mortars. This series of tests was analyzed by AFRLs Directed Energy Directorate, which substantiated predictions that a mortar could be destroyed at ranges greater than 550 meters. The laboratory and field testing anchored the high-fidelity directed energy weapon system effectiveness model." A particular attraction for this class of weapons is what the US Navy label the "deep magazine" effect - as long as electrical power is available to drive such a laser, it will be capable of engaging targets, unlike guns and missiles which are limited by munitions load, and chemical lasers, which are limited by unique fuel supply. | ||||||||||||
![]() | ||||||||||||
The interest in the use of High Energy Laser (HEL) Directed Energy Weapons (DEW) observed in the US, EU and Israel is paralleled by a development effort at Almaz-Antey aimed at trialling HEL DEW technology for air defence applications.
Little has been disclosed by Almaz-Antey on the detail of this program. It clearly intended to build up expertise and experience across the whole spectrum of necessary capabilities, in this instance beam director optics, adaptive optics, tracking capabilities, and high continuous wave power level laser designs. The laser depicted is a CO2 Gas Dynamic Laser (GDL), the same technology used by the US Air Force during the 1990s Airborne Laser Laboratory (ALL) program. It operates in the LWIR band at 10.6 microns, and is operationally attractive due to its simple fuel supply in comparison with Deuterium Fluoride (DF) and Chemical Oxygen Iodine Laser (COIL). What is less attractive about CO2 GDLs is that tropospheric CO2molecules increase propagation losses, and aluminium, the primary structural material in many potential targets, has a very high reflectance in this band, thus reducing power coupling efficiency into the target, and increasing dwell time. What has not been disclosed by Almaz-Antey is the progress on this project, especially in the critical area of adaptive optics and wavefront sensor technology for controlling adaptive mirrors. GDL technology is relatively mature, and derivative chemical laser designs will be largely determined by Russian capabilities in developing power modules for a given laser type. The choice of CO2 GDL may have been simply determined by its availability and low risk, as a means of demonstrating and proving other more sensitive system components. ![]() An operational HEL DEW air defence system will emerge only once the laser and beam director technology has matured to the point where a robust deployable design can be built. Given the Russian penchant for robustness and incremental evolution of designs, it is not difficult to postulate a configuration for such a system:
The CONOPS for such a system would be similar to the US Army MTHEL system, although it is likely the Russians will pursue a fully mobile configuration, consistent with their doctrine for SAM systems (refer below). It is likely that a key role of such a DEW would be the interception of PGMs, this placing the weapon system firmly in the domain of point defence.
Until we see further disclosures from the Russian MoD or Almaz-Antey, a more detailed assessment of this system is not feasible. Given the sensitivity of HEL weapon lethality performance to operating wavelength and beam quality, any predictions of achievable range performance would be at best speculative. For a system to be operationally effective, a sustained power output of the order of a MegaWatt would be required.
![]()
1987 concept illustration produced by the DIA, showing the expected configuration of the operational Soviet HEL DEW weapon system (US DoD).
| ||||||||||||
![]()
(Images RuMoD via testpilot.ru)
Initiated by the Soviets as a parallel program to the US Air Force Airborne Laser Laboratory, the Almaz/Beriev A-60 program aimed to demonstrate an airborne HEL DEW capability, and provide baseline data for the development of an operational weapon. The A-60 was therefore a research testbed, even if an operational capability were to be later based on this design.
Two demonstrators were built, the first flying in 1981, the second in 1991. Much of what is available from Russian open sources does not detail actual progress or achievements in this program. Observable design modifications to the Il-76MD Candid host airframe include:
All open source imagery shows a dummy optical beam director turret installation in the nose. Claims by Russian authors that the beam director was to be deployed from a dorsal hatch are impossible to reconcile with the unique ball turret shaping characteristic of a rotating and tilting beam director. Subject to basic design of the turret, this arrangement would provide at least hemispherical solid angle coverage, and possibly slightly more subject to turret tilt angle relative to the cylindrical fairing shroud.
There have been no disclosures on the type of laser intended for the A-60, although given the concurrent effort by Almaz on the CO2 GDL for the ground based mobile point defence weapon, it is reasonable to assume the same design. The large exhaust port in the aft fuselage is consistent with a GDL or chemical laser.
The current status of this program is not clear. At the end of the Cold War research funding collapsed for most advanced programs, but it is not known whether this project was mothballed or disbanded. If the Russian Air Force sought an airborne laser weapon then the A-60 project would be a viable starting point.
![]()
Boeing YAL-1A ABL beam director turret. The Soviets opted for this installation arrangement almost two decades before the YAL-1A program was defined (US DoD).
![]() ![]() ![]() | ||||||||||||
![]() | ||||||||||||
The is an abundance of material in various publications, especially web based, claiming a well developed and advanced High Energy Laser Directed Weapons program funded by the PLA. Unfortunately, much of this material lacks supporting evidence, and there are to date no unclassified images of PLA HEL DEW components, such as beam directors and laser power stages. What can however be tracked readily in the unclassified domain is research effort, via publications in Chinese language academic research journals, of which there is an abundance in the area of optics and laser physics. These publications provide some insight into the level of advancement in Chinese indigenous research into the basic physics of HELs and the basic technology required to construct HEL DEWs. A survey of unclassified journal publications in relevant technologies is very revealing, and shows that China does indeed have a major research program under way to develop state of the art HEL DEWs. In the area of high power lasers, research is being conducted in both Chemical Oxygen Iodine Lasers (COIL) and Free Electron Lasers (FEL). No less than 13 papers were found covering COIL physics, including topics such as performance modelling, the impact of water and nitrogen content on laser efficiency, and also detailing considerable effort in developing high efficiency atomic oxygen generators, critical to COIL performance. Free Electron Lasers, attractive as they are electrically powered and highly tunable, are another area of significant research effort in China, with no less than 25 papers found in an initial search. These cover a range of topics in basic FEL physics and design optimisation. This research overlaps with a research effort in non-nuclear X-ray laser technology, the latter including the design of X-ray beam splitters and other components required for practical applications. Chinese research is not confined to high power laser science and technology alone. A major research program is active in deformable mirror technology, especially using micromachined membranes (MEMS), and including claims of innovation in the technology. No less than 25 journal papers were found in this area. The other component critical to efficient long range high power laser weapons is the wavefront sensor, required for the control of the deformable mirror. No less than 40 papers were located, covering basic technology in Hartmann-Shack sensors, applications, modelling of atemospheric propagation, and measurement of beam quality. Much less visible is research in beam directors and fixed mirror components, with only one paper found, describing the design optimisation of a beam director comparable in size to that used in the US THEL program. What is evident from such a survey is that the PLA has the basic technology and research capabilities to design and build credible HEL DEWs. What cannot be assessed so easily is progress on the integration of these technologies to produce operationally viable weapons. However, given the possession of the component technologies, integration of these to produce a viable end product is only a matter of time and effort. A 1999 paper authored by US Army researcher Mark Stokes is cited with a claim that over "10,000 personnel -- including 3,000 engineers in 300 scientific research organizations -- with nearly 40 percent of China's laser research and development (R & D) devoted to military applications". Other public claims include the development of Deuterium Fluoride and Free Electron lasers (THEL/MIRACL), and a cruise missile defence capability based on HEL DEWs. The 2006 US DoD annual report to Congress on PLA modernisation also details a major effort in laser and RF directed energy weapons: "PRC officials have publicly indicated their intent to acquire RF weapons as a means of defeating technologically advanced military forces. Chinese writings have suggested that RF weapons could be used against C4ISR, guided missiles, computer networks, electronically-fused mines, aircraft carrier battle groups, and satellites in orbit. Analysis of Chinese technical literature indicates a major effort is underway to develop the technologies required for RF weapons, including high-power radiofrequency sources, prime-power generators, and antennas to radiate RF pulses. Chinese scientists are also investigating the effects of RF pulses on electronics and the propagation of these pulses through building walls and through the atmosphere. Furthermore, China appears to be assessing its own vulnerability to RF weapons and exploring ways to “harden” electronics. China is also involved in advanced, state-of-the-art research and development in laser technologies, including both low- and high-energy lasers. While much of China’s efforts are commercial in nature, the PLA and the government directly support some of this research, suggesting that discoveries or findings could be used to develop future laser weapons. Moreover, China has fielded in its own forces and marketed for sale abroad low energy laser weapons. Non-weapon military lasers are already widespread in the PLA." In evidence to Congress last year, the US DoD detailed the use of a PLA HEL to attack a low orbit US reconnaissance satellite. US sources have claimed that China made use of Russian expertise to advance their laser weapons effort. In summary, until the PLA unveils its first generation of HEL DEWs to global scrutiny, detailed assessments of capability will continue to be at best speculative. However, the PLA will be a major player in HEL DEWs and we can expect point defence applications such as counter-PGM and counter-cruise missile systems to be the first to emerge, as the power, beam quality and pointing requirements are the least challenging. | ||||||||||||
References
| ||||||||||||
Technical Report APA-TR-2008-0501
|
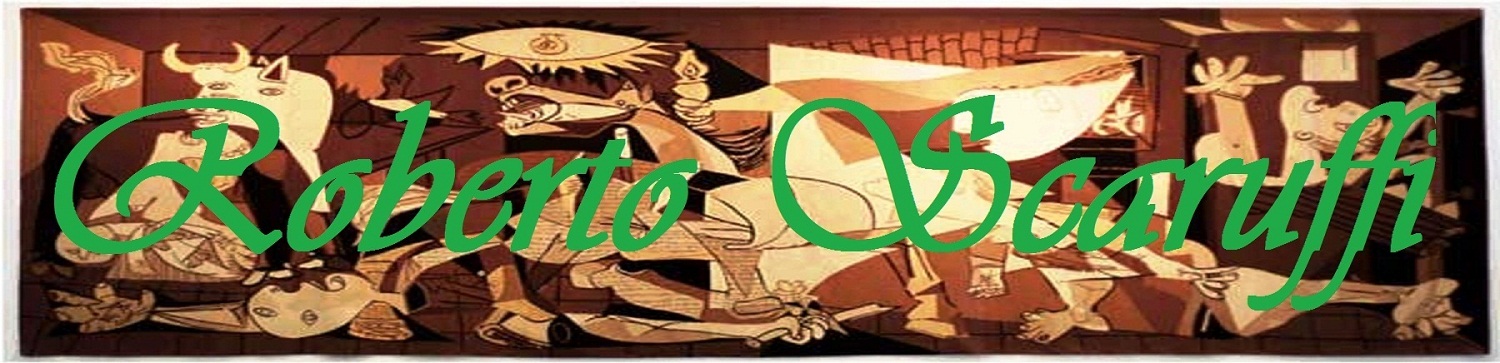
Everyday of Freedom is an Act of Faith for my writings ============> http://robertoscaruffi.blogspot.com for something on religions ===> http://scaruffi1.blogspot.com